Ein tiefer Einblick in Federn: Typen, Anwendungen und Auswahlhilfe Einführung in Federn
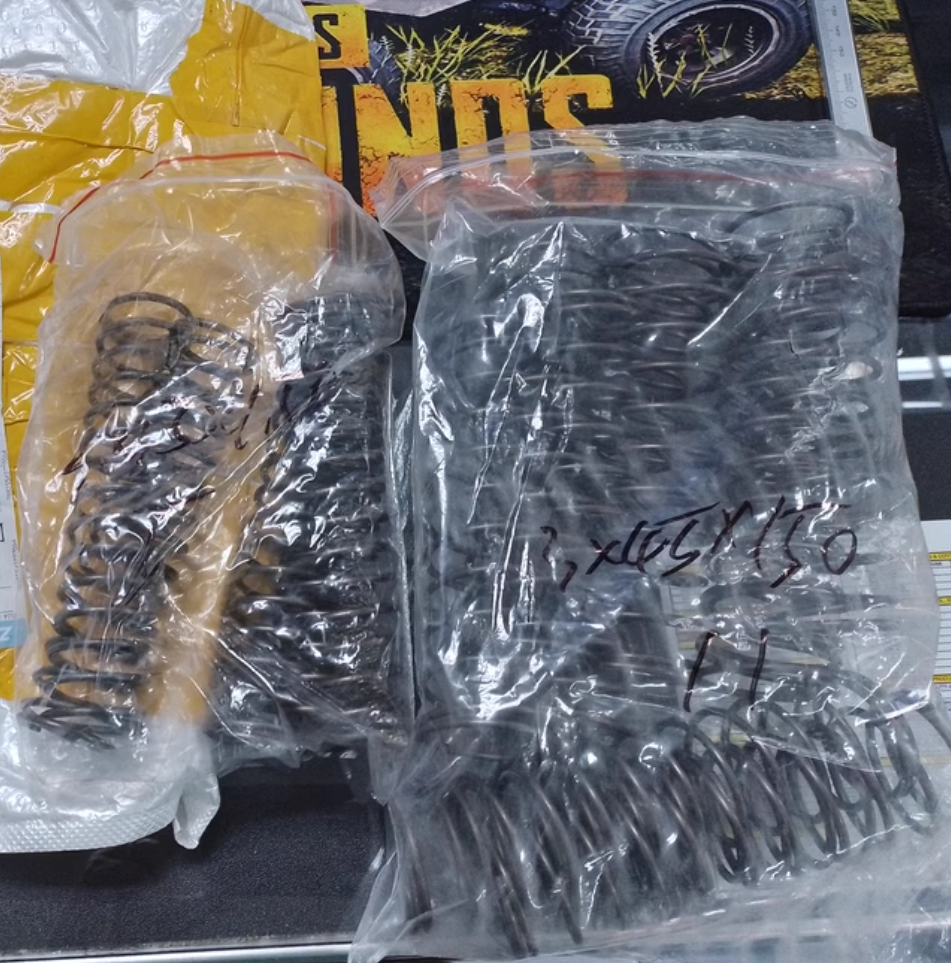
Federn im Detail: Typen, Anwendungen und Auswahlhilfe. Einführung in Federn. Eine Feder ist ein häufig verwendetes mechanisches Bauteil, das für seine einzigartigen elastischen Eigenschaften bekannt ist. Sie speichert und gibt Energie durch Verformung ab und spielt in verschiedenen Branchen eine entscheidende Rolle. Das Grundprinzip einer Feder ist ihre Fähigkeit, Energie durch elastische Verformung zu speichern und freizusetzen, wodurch das mechanische Verhalten in verschiedenen Systemen reguliert wird. Federn gibt es in verschiedenen Formen, darunter Druckfedern, Zugfedern, Torsionsfedern und andere spezielle Typen. Konstruktion und Materialauswahl einer Feder müssen die spezifischen Anforderungen ihrer vorgesehenen Anwendung berücksichtigen, um optimale Leistung und Haltbarkeit zu gewährleisten. Gängige Federtypen und ihre Anwendungen. Druckfedern. Druckfedern gehören zu den am häufigsten verwendeten Federtypen. Sie sind so konstruiert, dass sie Druckkräfte aufnehmen und beim Zusammendrücken Energie speichern und nach Wegfall der äußeren Kraft wieder in ihre ursprüngliche Form zurückkehren. Druckfedern finden sich häufig in Fahrzeugfederungssystemen, Bürogeräten (z. B. Stuhlfedern) und mechanischen Getrieben. In Branchen wie der Automatisierung und dem Maschinenbau spielen Druckfedern eine wesentliche Rolle für Stabilität und Präzision. Anwendungsbeispiele: Fahrzeugfederungssysteme: Druckfedern absorbieren Stöße von unebenen Straßenoberflächen und sorgen so für ein ruhiges Fahrgefühl. Druckluftwerkzeuge: In Druckluftwerkzeugen helfen Druckfedern, den Luftdruck zu regeln und die Start- und Stopp-Funktionen des Werkzeugs zu steuern. ZugfedernZugfedern sind für die Aufnahme von Zugkräften ausgelegt. Sie bestehen typischerweise aus gewickeltem Metalldraht und sind für ihre hohe Zugfestigkeit und Elastizität bekannt. Zugfedern werden in einer Vielzahl von Anwendungen eingesetzt, z. B. in Türschließmechanismen, Federwaagen, mechanischen Armen und Spielzeugen, insbesondere in Geräten, bei denen Komponenten nach der Dehnung in ihre Ausgangsposition zurückkehren müssen. Anwendungsbeispiele: Türschließmechanismen: Zugfedern werden häufig in automatischen Türen und Vorhangsystemen zur Kontrolle von Zugkräften eingesetzt. Federwaagen: Zugfedern werden in Federwaagen zur genauen Messung von Kräften oder Gewichten verwendet. TorsionsfedernTorsionsfedern speichern Energie durch Verdrehen und eignen sich daher für Anwendungen, die Drehbewegungen erfordern. Sie werden häufig in mechanischen Geräten und Werkzeugen eingesetzt, wo sie die Start-Stopp-Steuerung und die Drehmomentübertragung ermöglichen. Torsionsfedern sind für viele Präzisionsinstrumente und -geräte unverzichtbar, darunter Uhren, automatische Türschlösser und Federmotoren. Anwendungsbeispiele: Mechanische Uhren: Torsionsfedern sind das Herzstück des Antriebssystems mechanischer Uhren und liefern lang anhaltende Energie. Automatische Türschlösser: In vielen automatischen Türschlössern liefern Torsionsfedern das nötige Drehmoment zum Ver- und Entriegeln. Spezialfedertypen: Neben den gängigen Typen gibt es Spezialfedern wie Gasfedern und Rollfedern, die jeweils für bestimmte Anwendungen entwickelt wurden. Gasfedern werden aufgrund ihrer effizienten mechanischen Eigenschaften und Langlebigkeit häufig in der Automobil-, Möbel- und Luftfahrtindustrie eingesetzt. Rollfedern werden häufig in Anwendungen eingesetzt, die eine konstante Kraftabgabe erfordern, wie z. B. Rückstellfedern. Federkonstruktion und Fertigungsprinzipien: Bei der Konstruktion einer Feder müssen verschiedene Faktoren berücksichtigt werden, darunter mechanische Eigenschaften, Materialauswahl, Größenausführung, Tragfähigkeit und Arbeitsumgebung. Die Fertigungsprinzipien beinhalten Kenntnisse über Elastizität, Materialkunde und Verarbeitungstechnologien. Zu den gängigen Konstruktionsprinzipien gehören: Hookesches Gesetz: Beschreibt die lineare Beziehung zwischen der von einer Feder ausgeübten Kraft und ihrer Auslenkung innerhalb der Elastizitätsgrenze. Das Hookesche Gesetz wird mathematisch als F = kx ausgedrückt, wobei F die Kraft, k die Federkonstante und x die Auslenkung ist. Ermüdungslebensdauer und Spannungsanalyse: Federn unterliegen mit der Zeit Ermüdungsspannungen. Deshalb ist eine Konstruktion mit dauerhafter Zuverlässigkeit unabdingbar. Eine geeignete Spannungsanalyse gewährleistet, dass die Feder während ihrer erwarteten Lebensdauer gut funktioniert. Materialauswahl: Das für eine Feder gewählte Material bestimmt ihre Festigkeit, Elastizität, Korrosionsbeständigkeit und andere wichtige Eigenschaften. Gängige Materialien sind Kohlenstoffstahl (z. B. SAE 1060, SAE 1070), Edelstahl (z. B. AISI 302, AISI 304) und legierte Stähle (z. B. Federstahl). So wählen Sie die richtige Feder aus Bei der Auswahl einer Feder sollten mehrere wichtige Faktoren berücksichtigt werden: Materialauswahl: Wählen Sie Materialien, die für die Arbeitsumgebung und die Belastungsanforderungen geeignet sind. Gängige Federmaterialien sind Kohlenstoffstahl (z. B. SAE 1060, SAE 1070), Edelstahl (z. B. AISI 302, AISI 304) und legierter Stahl (z. B. Federstahl). Die Auswahl der Materialien richtet sich nach ihrer Belastbarkeit, Korrosionsbeständigkeit und Temperaturextremen. Größe und Spezifikationen: Definieren Sie die erforderlichen Federabmessungen wie Drahtdurchmesser, Innen- und Außendurchmesser, freie Länge und Steigung klar. Auch Form und Aufbau der Feder (z. B. Spiral- oder Flachfeder) müssen der jeweiligen Anwendung angepasst werden. Belastungsanforderungen und Federkonstante: Wählen Sie je nach den Kräften, die die Feder bewältigen muss, eine Feder mit der entsprechenden Federkonstante (k-Wert) und Festigkeit. Wählen Sie Federtyp und -abmessungen passend zur erforderlichen Verformung und Tragfähigkeit. Arbeitsumgebung: Die Einsatzumgebung der Feder beeinflusst ihre Leistung und Lebensdauer. Beispielsweise erfordern Federn, die in Umgebungen mit hohen Temperaturen, hoher Luftfeuchtigkeit oder chemisch korrosiven Einflüssen eingesetzt werden, spezielle Materialien wie hitzebeständige Stähle oder korrosionsbeständige Legierungen. Qualitätskontrolle: Ein hochwertiger Federhersteller führt ein strenges Qualitätskontrollsystem ein, um sicherzustellen, dass jede Feder die Konstruktionsspezifikationen erfüllt und zuverlässig funktioniert. Standardisierte Fertigungsprozesse und strenge Prüfmethoden sind der Schlüssel zur Sicherstellung der Produktqualität. Fazit: Federn spielen als wichtige mechanische Komponenten eine entscheidende Rolle im täglichen Leben sowie in der industriellen Produktion, der wissenschaftlichen Forschung und der Herstellung moderner Geräte. Von der traditionellen Druckfeder bis zur komplexeren Torsionsfeder hat jeder Federtyp seinen einzigartigen Anwendungswert. Bei der Auswahl einer Feder sollten Faktoren wie Material, Größe, Belastung und Arbeitsumgebung sorgfältig berücksichtigt werden, um optimale Leistung und eine längere Lebensdauer zu gewährleisten. Als professioneller Federhersteller bieten wir eine breite Palette an Federprodukten und kundenspezifischen Lösungen, um unsere Kunden bei der Auswahl der am besten geeigneten Federn für ihre spezifischen Anforderungen zu unterstützen. Für weitere Informationen oder zum Kauf von Federn besuchen Sie unseren Online-Shop und erhalten Sie kompetente Beratung.
Applicable Scenarios For Torsion Springs
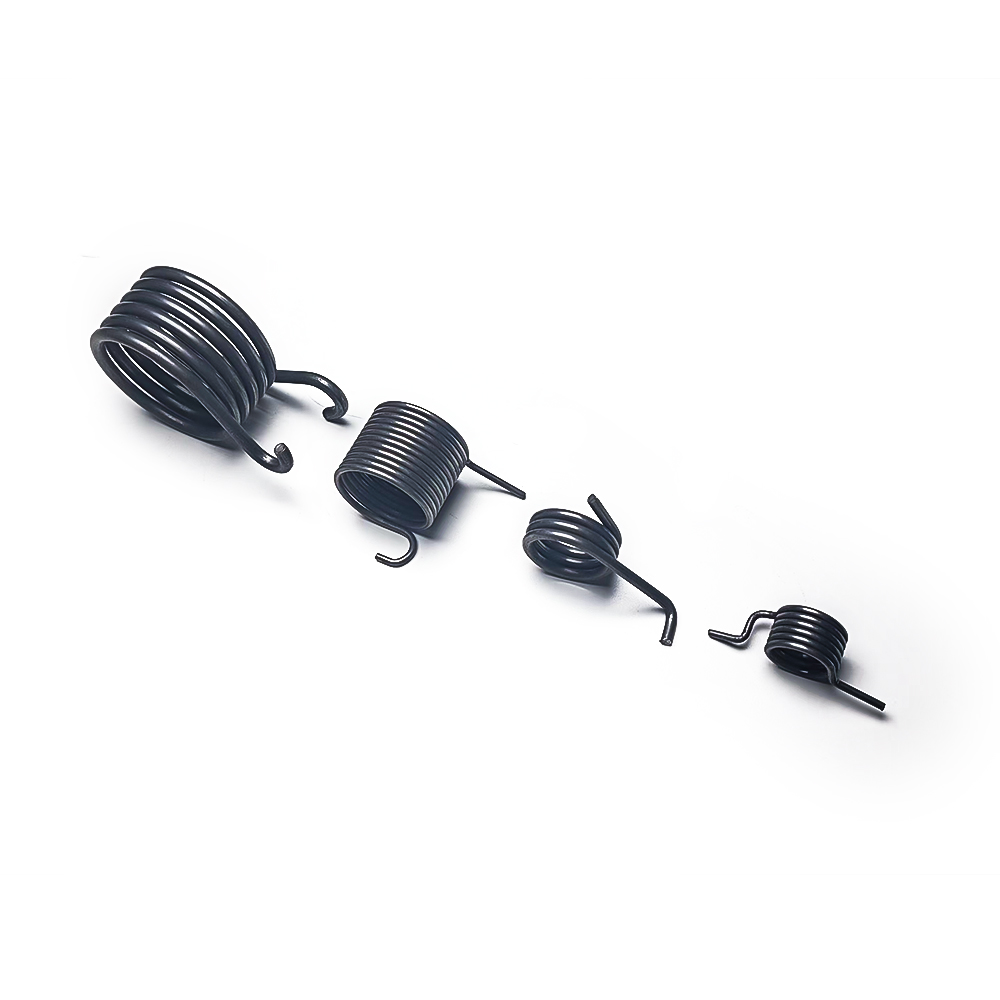
Service Details Torsion springs Applicable scenarios for torsion springs Torsion springs are the components that upon twisting, exert a force in the opposite direction. This force is directly proportional to the twisting. When twisted, these springs store mechanical energy. When released, these springs come back to their original position. The torsion springs are helical shaped springs that are capable of producing torsional or rotational forces. Based on the application for which they are used, these springs are either clockwise or anti-clockwise wound. Thus, depending on its use, these springs have a capability to work in both the directions. Where are the torsion springs used? Read the post to find out the uses of torsion springs in different applications. Top 5 Applications of Torsion Springs There are a number of applications, where torsion springs find their use. Here are some of the major areas of its application: Clocks: Torsion springs are used in torsion pendulum clocks. In such clocks, a torsion spring is used to suspend a wheel-shaped weight from the center of the clock. As the weight rotates around its axis, it twists the spring. As per its nature, the spring exerts an equal opposite force on the wheel and makes it twist in the other direction. Thus, there is a continuous back and forth motion of the pendulum, which makes the clock work by driving the gears to which it is attached. Clothes Pins: This is one of the most common applications of these springs. The working of clothes pins is facilitated by the torsion springs. These springs provide an excellent clamping action, which is why they are used in this application. Automotive: Torsion springs are known for providing even tension, along with smooth and frictionless motion. This is the reason why these springs are widely used in the automotive industry for various parts such as a vehicle suspension system, chassis, automotive valves, clutches, and gear shifters. These springs are made from materials like chrome silicone, which makes it resistant to environmental abuse, along with providing it additional strength. Medical Equipment: In the medical industry, the torsion springs are used in a number of complex equipment. Different medical equipment that make use of these springs include medical immobilization devices, hospital beds, several dental applications, wheelchair lifts and many more. For additional strength, these springs are made from high carbon steel, which also helps increase the life span of the spring. Door Hinges: These springs are widely used in different types of door hinges. Right from automobile doors and residential doors to heavy duty doors at warehouses, torsion springs are used in the hinges. These springs allow the door to come back to its original position. You must have noticed the doors in saloons that move back and forth when opened, but ultimately become stationary at their original position. This is due to the springs used in their hinges.
Introduction And Working Principle Of Compression Spring
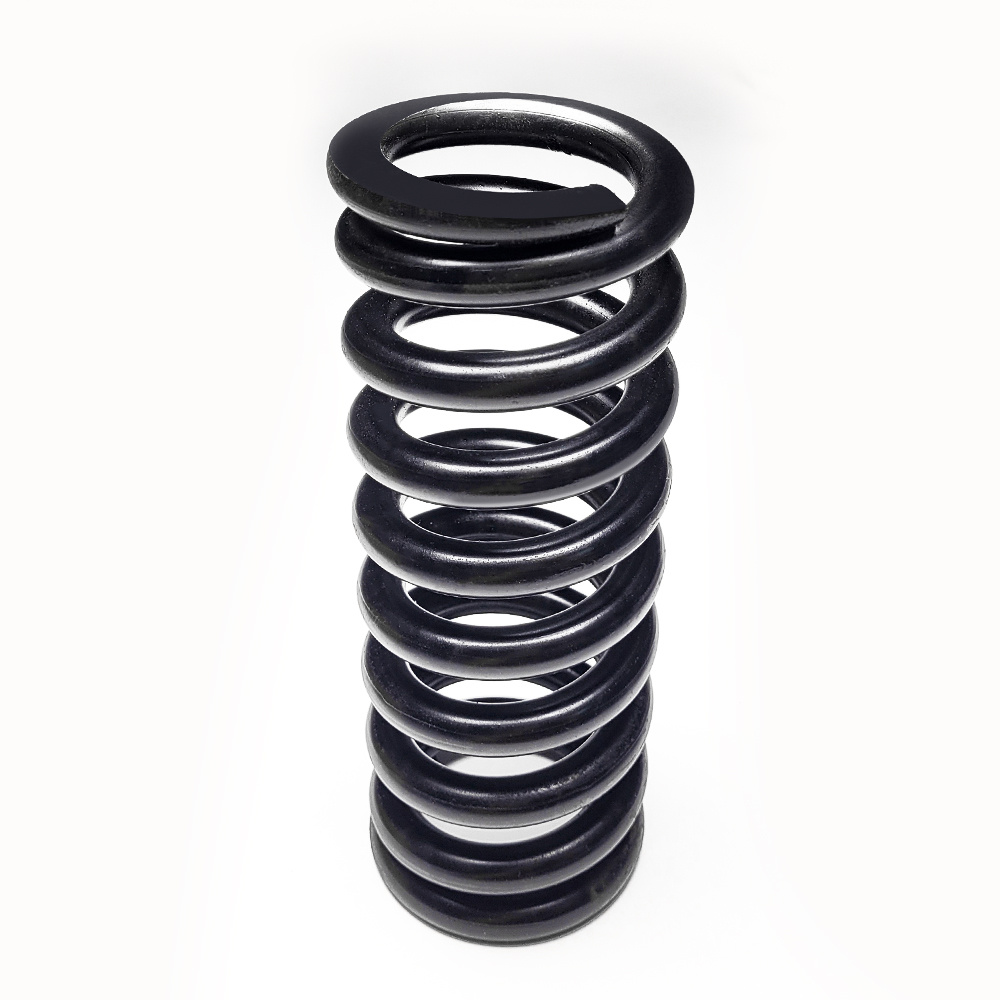
compression spring Introduction and working principle of compression spring Compression Springs are open-coil helical springs wound or constructed to oppose compression along the axis of wind. Helical Compression is the most common metal spring configuration. These coil springs can work independently, though often assembled over a guide rod or fitted inside a hole. When you put a load on a compression coil spring, making it shorter, it pushes back against the load and tries to get back to its original length. Compression springs offer resistance to linear compressing forces (push), and are in fact one of the most efficient energy storage devices available. The amount of energy stored in a compression spring is determined by the spring’s material properties, wire diameter, and number of coils. The spring’s rate, or stiffness, is determined by the wire diameter and the number of coils. The spring’s rate is the change in force per unit change in length, and it is measured in pounds per inch or newtons per millimeter. The spring’s rate can be adjusted by varying the wire diameter or the number of coils. Compression Spring Applications Compression Springs are found in a wide variety of applications ranging from automotive engines and large stamping presses to major appliances and lawn mowers to medical devices, cell phones, electronics and sensitive instrumentation devices. The most basic installation is anywhere requiring a push button. Conical type springs are generally used in applications requiring low solid height and increased resistance to surging. Key Compression Spring Parameters Rate: Spring rate is the change in load per unit deflection in pounds per inch (lbs/in) or Newtons per millimeter (N/mm). Stress: The dimensions, along with the load and deflection requirements, determine the stresses in the spring. When a compression spring is loaded, the coiled wire is stressed in torsion. The stress is greatest at the surface of the wire; as the spring is deflected, the load varies, causing a range of operating stress. Stress and stress range govern the life of the spring. The wider the operating stress range, the lower the maximum stress must be to obtain comparable life. Relatively high stresses may be used when the operating stress range is narrow or if the spring is subjected to static loads only. Outside Diameter: The diameter of the cylindrical envelope formed by the outside surface of the coils of a spring. Hole Diameter: This is a measurement of the space where you would insert a compression spring. It is the diameter of a mating part to a compression spring and often commonly mistaken for a dimension of the spring itself. The hole diameter should be designed larger than your compression spring’s outside diameter factoring tolerance and spring expansion under load. Rod Diameter: This is a measurement of the rod that goes through the inside of a compression spring. Essentially a mating part, this rod can work as a guide shaft to minimize spring buckling under load. The rod diameter should be designed smaller than your compression spring’s inside diameter factoring tolerance; however, not too small or else it loses ability to minimize spring buckling. Free Length: The length of a spring when it is not loaded. NOTE: In the case of extension springs, this may include the anchor ends. Wire Diameter: This is a size measurement of the raw material used to form a spring. Conventional springs are made with round wires that are specified to a diameter. Consult our guide on How to Measure a Compression Spring. Solid Height: This is a length dimension of a compression spring at its maximum loaded condition. Effectively, this is the compression spring’s height when all the coils are pressed together. Spring Set: This is an occurrence when a spring is loaded beyond its material elastic strength. It is a kind of permanent deformation that is noticeable when a spring does not return to its original length after releasing a deflection load. Depending on the application, spring set can be either desirable or undesirable. Load at Solid Height: This is a measurement of the force required to completely deflect a compression spring to where the coils are fully pressed together. For product designers that want to avoid the occurrence of bottoming out a compression spring, Load at Solid Height is quick reference property to find springs capable of handling an assembly’s maximum operating load. Resources
Stainless Steel Grades: All About 304

Stainless Steel Grades: All About 304 Steel is one of the most widely used materials in the world, useful for creating an infinite variety of different structures and components. Primarily composed of carbon and iron, steel can be further alloyed with a range of other elements to create a vast family of steel types. One of these elements is chromium: when chromium is added to steel, it results in stainless steel. Within the stainless steel family, there are numerous different types and grades. By far the most common grade is 304, which accounts for more than 50% of the stainless steel used around the world. 304 grade stainless steel is austenitic, a term which relates to its molecular structure and signifies that nickel has been added to the alloy. Austenitic stainless steels are the most common; as much as 70% of commercially-produced stainless steel is of the austenitic type. Stainless steel of grade 304 is composed primarily of iron, which makes up between 66% and 75% of its composition. Also included in the alloying mix is between 18% and 20% chromium and between 8% and 10.5% nickel. With a density measured at around eight grams per cubic centimetre, 304 stainless steel may also contain trace amount of other elements like silicon, sulphur , phosphorus and manganese. As standard, 304 stainless steel has around 0.08% carbon content. 304H grade has a higher carbon content of up to 0.1% while 304L has a lower amount of carbon at around 0.03%. As higher carbon content improves the strength of stainless steel, 304H is widely used in high-temperature applications. 304L, with its lower carbon content, displays increased ductility and so is often used for welding components. Austenitic stainless steels like 304 have a yield strength that represents a comparatively low proportion of its tensile strength: between 40% and 45%. Its yield strength can be further improved when the material is cold-worked. This is particularly useful when making things like spring wire. Salient qualites of 304 grade stainless steel include: Good corrosion resistance No magnetism when the metal is annealed An ability to be work-hardened Exceptional ductility and weldability High level of hygiene Ease of cleaning Good performance at both high and low temperatures Share the Post: Related Posts